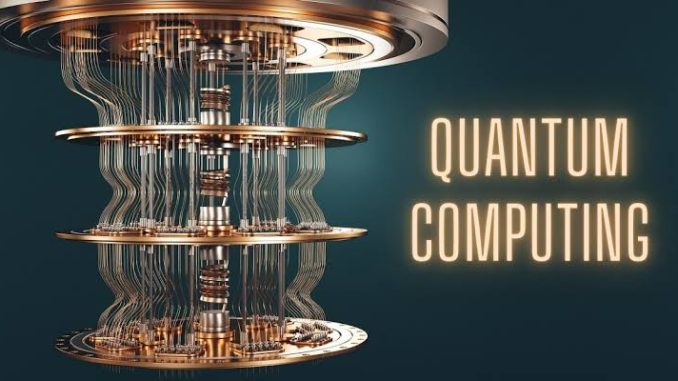
Quantum computing stands at the forefront of technological innovation, promising to revolutionize computation by harnessing the principles of quantum mechanics to perform calculations at speeds and scales that were once thought impossible. While the potential of quantum computing to solve complex problems and drive scientific breakthroughs is vast, it also presents unique challenges and considerations that must be addressed to realize its full potential. In this article, we explore the promise and perils of quantum computing, examining its applications, implications, and the road ahead for this transformative technology.
At its core, quantum computing leverages the principles of quantum mechanics, such as superposition and entanglement, to perform calculations in ways that traditional computers cannot. Unlike classical computers, which use bits to represent data as either 0 or 1, quantum computers use quantum bits, or qubits, which can exist in multiple states simultaneously, allowing for parallel computation and exponential increases in processing power.
One of the most promising applications of quantum computing is in the field of cryptography and cybersecurity. Quantum computers have the potential to break many of the cryptographic algorithms that currently secure the internet and protect sensitive information, such as RSA and ECC encryption. This poses a significant threat to cybersecurity and data privacy, as quantum computers could theoretically decrypt encrypted data and compromise secure communication channels.
However, quantum computing also offers solutions to cybersecurity challenges by enabling the development of quantum-resistant cryptographic algorithms that are immune to attacks by quantum computers. Researchers are actively exploring post-quantum cryptography techniques, such as lattice-based cryptography, hash-based cryptography, and code-based cryptography, to develop encryption schemes that are secure against quantum attacks. Moreover, quantum key distribution (QKD) protocols leverage the principles of quantum mechanics to enable secure communication channels that are resistant to interception and eavesdropping.
Moreover, quantum computing has the potential to revolutionize fields such as drug discovery, materials science, and optimization by solving complex problems that are intractable for classical computers. For example, quantum computers can simulate the behavior of molecules and atoms with unprecedented accuracy, enabling researchers to design new drugs, catalysts, and materials with desired properties and characteristics. Moreover, quantum algorithms such as Grover’s algorithm and Shor’s algorithm offer exponential speedups for optimization and factorization problems, which have broad applications in fields such as logistics, supply chain management, and financial modeling.
Furthermore, quantum computing has the potential to accelerate scientific discovery and innovation by enabling researchers to tackle some of the most pressing challenges in physics, chemistry, and engineering. For example, quantum computers can simulate the behavior of complex quantum systems, such as high-temperature superconductors or exotic materials, which are difficult or impossible to study using classical computational methods. Moreover, quantum machine learning algorithms can analyze large datasets and extract insights that would be impractical for classical computers, enabling advances in fields such as climate modeling, genomics, and particle physics.
Despite its promise, quantum computing also presents unique challenges and considerations that must be addressed to realize its full potential. One of the biggest challenges facing quantum computing is decoherence, which refers to the loss of quantum coherence and the resulting errors in computation. Quantum systems are highly sensitive to external disturbances and noise, which can cause qubits to lose their quantum state and introduce errors into calculations. Overcoming decoherence requires advances in qubit coherence times, error correction techniques, and fault-tolerant quantum computing architectures.
Moreover, quantum computing requires significant infrastructure and resources, including specialized hardware, cryogenic cooling systems, and sophisticated control and measurement techniques. Building and maintaining quantum computers is costly and technically challenging, limiting access to quantum computing resources and expertise. Moreover, quantum computing applications require specialized algorithms and software development skills, which are currently in short supply and require investment in education and training programs.
Furthermore, quantum computing raises ethical and societal considerations regarding its potential impact on privacy, security, and inequality. The ability of quantum computers to break encryption schemes and compromise secure communication channels poses risks to data privacy and cybersecurity, raising concerns about the need for robust encryption standards and regulations. Moreover, the unequal access to quantum computing resources and expertise could exacerbate existing disparities in access to technology and opportunities, widening the digital divide and exacerbating social inequalities.
In conclusion, quantum computing holds immense promise for solving complex problems and driving scientific and technological innovation across industries. From cryptography and cybersecurity to drug discovery, materials science, and optimization, quantum computing has the potential to revolutionize the way we compute, communicate, and innovate. However, realizing the full potential of quantum computing requires overcoming technical challenges, addressing ethical and societal considerations, and fostering collaboration and investment in research, education, and infrastructure to advance this transformative technology.